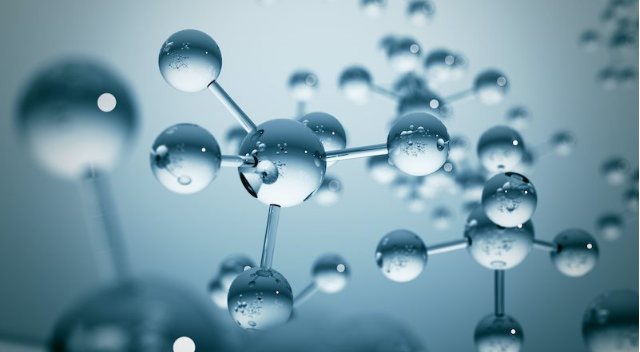
Hydrocarbons can be found almost everywhere. When most people hear the name of this group of compounds they tend to connote it solely with fuels, whereas that is not entirely accurate. Take clothing, for example, 60% of it worldwide contains hydrocarbons1. But that is not as much of a surprise when we realise that products such as crayons, lipstick, disposable ball-point pens2, training shoes, life jackets3, toothpaste and many more contain hydrocarbon derivatives4. Moreover, we even eat hydrocarbons! There is a very high chance the very cereal I ate the day I wrote this essay contained a member of this precise group of compounds5. So why are they everywhere? The reason lies in the source of hydrocarbons, namely: natural gas, crude oil and coal. Throughout the history of mankind loads of applicationshave been foundfor them. They have been incorporated into the processes of nearly every industry.
There are two different types of hydrocarbons: saturated and unsaturated hydrocarbons. Saturated hydrocarbons contain solely single bonds while unsaturated ones contain at least one multiple bond between carbon atoms. This diversity results in a further grouping: alkanes, alkenes and alkynes. Alkanes are hydrocarbons of solely single bonds between carbon atoms. Alkenes are hydrocarbons, containing at least one double-bond between carbon atoms and alkynes - containing at least one triple-bond between carbon atoms6. In the following essay, I will take a closer look at how the representatives of these homologous groups shape what can be found around us.
I would like to begin with alkanes. They can be obtained through the distillation of natural energy resources - the previously mentioned; natural gas, crude oil and coal. Another method for alkane’s obtainment is possible due to their structure and it is called cracking. In this process, long alkane chain’s single bonds are torn to form a couple of shorter alkanes. Nevertheless, alkanes are the basic products of the distillation of energy resources, thus them being used in the fuel industry as, for instance, petroleum for cars, jet fuel, etc. makes perfect sense. Both petroleum and jet fuel are liquids. However, alkanes can also be present in gaseous and solid states of matter. This physical property comes down to a simple rule. A single alkane particle in liquid state of matter contains 5 to 16 carbon atoms, while a gaseous alkane particle - 1 to 4 carbon atoms and a solid alkane particle - 17 or more. Gaseous hydrocarbons include methane. One of its real-life uses is the generation of heat and electricity. Another interesting application of methane is rocket propulsion. Some tests utilising it had already been run and proved it to be usable. It is said that methane in liquid form may soon replace the traditional kerosene-based fuel. Just recently on May 5th Elon Musk’s company SpaceX successfully launched and landed a liquid methane propelled Starship prototype7. There are also solid alkanes that have their own appliances in real life. A mix of them can be found in paraffin wax which is used in candle production. Most alkanes have rather low reactivity due to their single chemical bonds of low energy. While it makes them more stable it doesn’t allow them to undergo addition reactions and polymerisation.
That is not the case for alkenes which have at least one double chemical bond. This results in their increased reactivity and, within the context of appliances, versatility. Since this is a chemistry essay I would like to examine at least one chemical process in detail. The action that particularly intrigued me was plastic production. Thus I’ve dived into the depths of the internet in order to try and analyse it on a molecular level. What is more, this process is one of the primary reasons why hydrocarbons are so widespread in the first place. Without further ado let me begin. In the very beginning, raw materials are refined into ethane and propane. Those compounds are exposed to the process of cracking8. The resulting products are ethylene and propylene. On a molecular level, these processes in terms of ethane look in the following way: ethane molecules (C2H6) are heated at high temperatures to break their single bonds and cause two hydrogen atoms to break off9. This results in the formation of ethylene (C2H4) and a hydrogen molecule (H2)10. What follows is polymerisation. Let us follow up on the ethane conversion to illustrate this step: the previously obtained ethylene molecules create chains through the breaking of their double chemical bonds. This is facilitated by specific temperatures, pressure and the presence of a catalyst. The resulting compound in terms of our ethane conversion example is called polyethylene. The last step that affects the final composition of the material is the compounding, where other compounds are mixed with ethylene and propylene. They are then shaped into pellets of material or final product11.This process highlights hydrocarbons’ (both alkanes and alkenes) role in the manufacturing industry. Some objects formed in such a way are packaging, wiring insulation and toys. However, alkenes not only take part in the process of plastic production. The previously mentioned ethene, for instance, also has the unique property of ripening fruits, thus it is utilised in ripening facilities to bring imported fruits to perfect shape before being sold.
There is one more group of hydrocarbons that I have not yet considered: alkynes. Like alkenes, they are also unsaturated hydrocarbons, but they contain at least one triple bond instead of a double. One of the most interesting uses of an alkyne - acetylene is the cutting and welding of metals. The metal is brought to a specific temperature at which it can burn on contact. It is then treated with an acetylene-oxygen mixture in the form of an extremely hot flame of 3200 degrees. The metal is instantly molten. This particular member of alkynes has another interesting property, when burned with the correct amount of air it gives a pure, white light. In the past, this came in handy in mines and other places where workers couldnot always use electrical bulbs and so relied on portable acetylene lamps instead. In present times, however, the compound is better known for being a key agent in the process of manufacturing many important organic compounds such as acetic acid, acetaldehyde and ethyl alcohol.
The above-listed compounds belong to a group labelled as hydrocarbon derivatives - compounds that are based on hydrocarbons but contain one or more hydrocarbon atoms substituted with other atoms or functional groups. Let’s take a look at their applications in real life. Acetic acid is a food additive, a solvent for resin and paints, it is utilised in pesticide, rubber and plastic production as well as printing processes12. Acetaldehyde is used to produce other chemicals but most interestingly it can be found in drugs and perfumes. When it comes to ethyl alcohol it is worth mentioning that, as the name suggests, it is a part of a hydrocarbon derivatives sub-group called alcohols - compounds whose molecules are composed of an alkyl group and a hydroxyl group -OH,as a functional group. Either way, ethyl alcohol has a particularly interesting application in real life for me. There is an acclaimed race car driver called Ken Block known for his “Gymkhana” YouTube series. Me being interested in all things cars, I had to find out more about how his machines operate. Interestingly, it turns out there is a lot of chemistry involved there. I have learned that some of his most extreme vehicles utilise a mixture of ethanol and methanol as special high-performance fuel13. The biggest advantage of methanol-based fuel is that as long as the car’s power unit can burn it fast enough, it outputs better potential power. It also “burns cooler” meaning it requires less heat energy to change from its liquid state to its vapour state and then power the engine14. Since overheating is less likely to occur this is a huge plus for methanol in comparison to gasoline. However, there are drawbacks, (if there weren’t any it would probably already be used widespread commercially). It is perhaps most visible from the pure chemistry standpoint: methanol is highly poisonous. Inhaling or consuming as little as 10 ml can cause permanent health damage - loss of sight and at 60 ml - even death. It also burns with a flame nearly invisible to the human eye so one can find himself at risk, not even realising a fire-related engine failure. These are the two primary factors holding back manufacturers from going 100% methanol and ethanol-based. The previously mentioned greater potential power output goes hand in hand with faster burning of fuel if one is opting for maximum performance. If it is to burn faster, there must be a different air to fuel ratio, thus a larger amount of methanol is required to make power. All in all, as for now, I believe methanol and ethanol-based fuels will continue to develop in the race car sphere. Once it will be optimised for safer usage and efficiency it might be used in mass production road vehicles.
To sum up, hydrocarbons and their derivatives can be found nearly everywhere. They have been incorporated into countless processes across energy, fuel, electricity, space, chemical, manufacturing, food, pharmaceutical, construction, car industries and many more. The resulting products shape what is around us. I was genuinely surprised by the extent to which the modern world relies on hydrocarbons. It makes it that much more pressing for us to find substitutes for them since they are based on fossil fuels. These, in turn, as we all know are starting to run out. Only new, improved materials from renewable sources can ensure a sustainable future for the upcoming generations.
Bruno Bugla, Edited by Aleksandra Kucharska
Comments